What my 16-month-old neuroscientist can teach you about learning
The path to mastery depends on failing. Watching my daughter learn to walk taught me a lot.
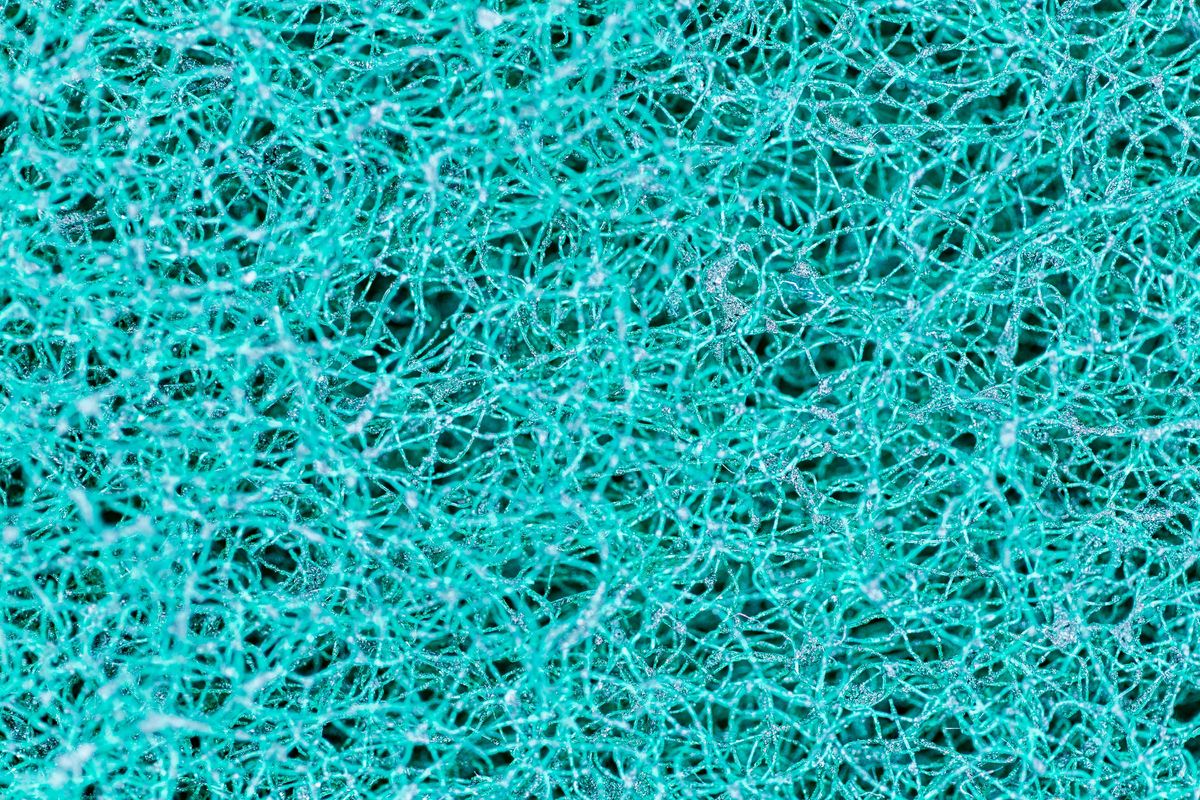
Her feet were firmly rooted in place, a bit more than shoulder-width apart. Her knees had a soft bend, and arms held out to her sides. She looked like she was about to surf the biggest wave of her life. She looked at me like, well now what?! My daughter, Everly, was just over 14 months old. She had been a very mobile infant but hadn't yet found a need to start walking. That was changing, though. She looked over at me, eyes wide, rocked to her left, and plodded her right foot forward. Success! She looked at me and held her mouth open like she couldn't believe what she was doing. She took another step forward and fell to her butt. In other words, she stuck the landing.
Since that day a little more than a month ago, Everly has become so proficient at walking it's almost an afterthought. It's hard to imagine a time when she wasn't walking at this point. Watching a child develop from a newborn to an infant to a toddler is an incredible thing. You're an observer of an explosion of physical and cognitive skills that you otherwise take for granted - chances are you don't remember what it was like yourself to have started talking or walking or brushing your teeth. Having studied the brain and the mind at University, it's been particularly remarkable to watch my child go through that process. It's given me both a real-life example to understand better what I've studied and has shown me how I can use those same principles to teach others better and continue down a path of learning myself.
Meandering is the Path
Everly is all about the scenic route. Her favorite path to get to the kitchen is typically a series of loops, turnabouts, and pit stops at all her favorite viewpoints from the living room through our dining area. She'll often stop midway, make a wide circle around my desk, walk over to a window overlooking our patio, watch some squirrels, toss a ball out of the way, then trot over to her table to grab her panda bear. The destination is never in doubt, but she always seems open to a new means of getting there. What seemed at first like distractibility, I now understand, is exploration - play really - that is just as, if not more important, than the process of getting from A to B.
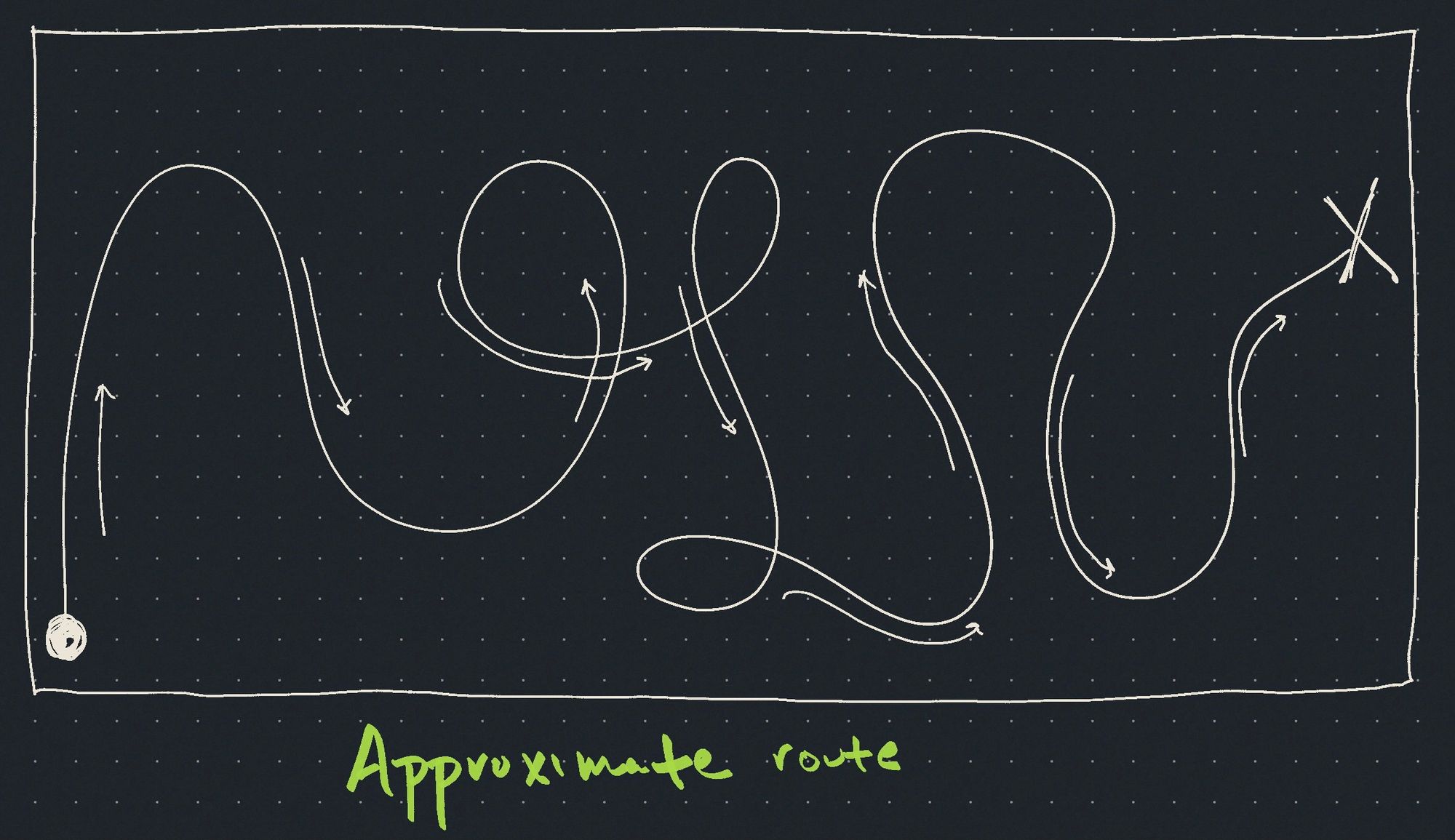
The importance of play in learning has been studied. In the book Beginners, Tom Vanderbilt highlights research from the NYU Infant Action Lab, which shows infants seem to be just as interested in walking for the sake of walking as they are in walking to get to something or somewhere. They aren't so much motivated by the utility of walking in and of itself as we often think. Infants seem to walk just as much in an empty room as they do in a room filled with toys. Watching Everly, at times, makes me feel like I want to help her - hold her hand to say, "Hey, if you just want to get your cup, let's just go get it." In part, raising children is as exhausting as it is because of the spontaneity and exploration it requires. The struggle is what's important, though. It would be easier to learn to walk by just walking from A to B over and over. Repetitions. Though the tradeoff for immediate success is a lack of resiliency - the moment an obstacle or new problem is put in front of Everly - she'd lack the prior experience to derive a new solution at the moment. Instead, by learning to walk through apparent randomness, Everly is using variability to her advantage. Cognitive psychologists call this "interleaving," an approach to skill development that leads to more robust performance over time. Rather than the monotonous practice of one skill, the loops, turns, stops, and detours are her practicing. She isn't just walking; she's turning, bending, twisting, and stepping. The scenic route is her cross-training.
In Range: How Generalists Triumph in a Specialized World, David Epstein explores the concept of mixed practice. I found particularly interesting the role of diverse experiences and their implications on the mental models we develop. Epstein writes, "..the more contexts in which something is learned, the more the learner creates abstract models, and the less they rely on any particular example. Learners become better at applying their knowledge to a situation they've never seen before, which is the essence of creativity."
To understand how this might play out for Everly as she's learning to walk, I think it's helpful to know how the body moves through space. On a general level, the brain has a particular area called the motor cortex. Its primary role is to receive input from other brain areas, integrate that information, and generate signals to the muscles in our arms, trunks, and legs to move us where we intend to go.
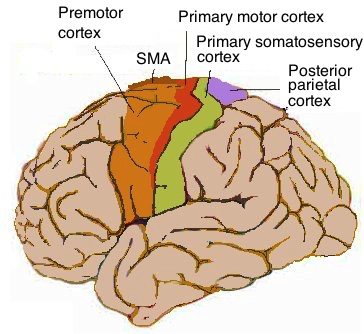
The motor cortex has two closely associated areas called the pre-motor cortex (PMC) and supplementary motor area (SMA). The PMC is responsible for responding to external stimuli and the SMA internal stimuli. That is, the PMC helps us navigate new, complex movements in response to new problems - obstacles in our path, a new exercise, or performing a medical procedure we've never done. The SMA uses the knowledge and experience we already have to help us carry out learned, well-rehearsed movements. For a given skill, activity between these two areas will shift from relying on the PMC to using the SMA as something becomes more familiar. The "enjoying the journey" mentality that infants take likely arms them with skills and experience that helps them be more successful at other, seemingly unrelated skills. In Beginners, Vanderbilt also describes prior research that looked at kids who took swim lessons, then analyzed their motor skills across different domains. They didn't just improve at swimming but also had improved hand-eye coordination and grasping compared to kids who didn't take swim lessons. Learning new skills teaches you lessons you can apply to new problems. The brain's PMC and SMA have a lot to do with that.
Everly prepares herself for breakthroughs by being open to taking a path that she may not have intended to go down. She doesn't see the objective as "I walk to get somewhere." Instead, she just walks.
Small Wins are Big Gains

In the mid-1800s, a cognitive psychologist named Edward Thorndike conducted a series of experiments looking at how cats seemed to learn. He would place a cat in a wooden box built with a switch on the box floor that would open a door when the switch was activated. The cats would wander around the box until they accidentally hit the switch that opened the door and could get out. To sweeten the deal, Thorndike would reward the animal (food) after having escaped. He noticed that the duration of time to hit the switch would decrease as a cat was placed in the box repeatedly - they were learning the association between the switch and escape. Thorndike was able to plot the time it took for the animals to understand this association - an S shape curve that became known as the "learning curve." It showed that animals learn the same way, gradually, through experience and that some animals learned more quickly than others.
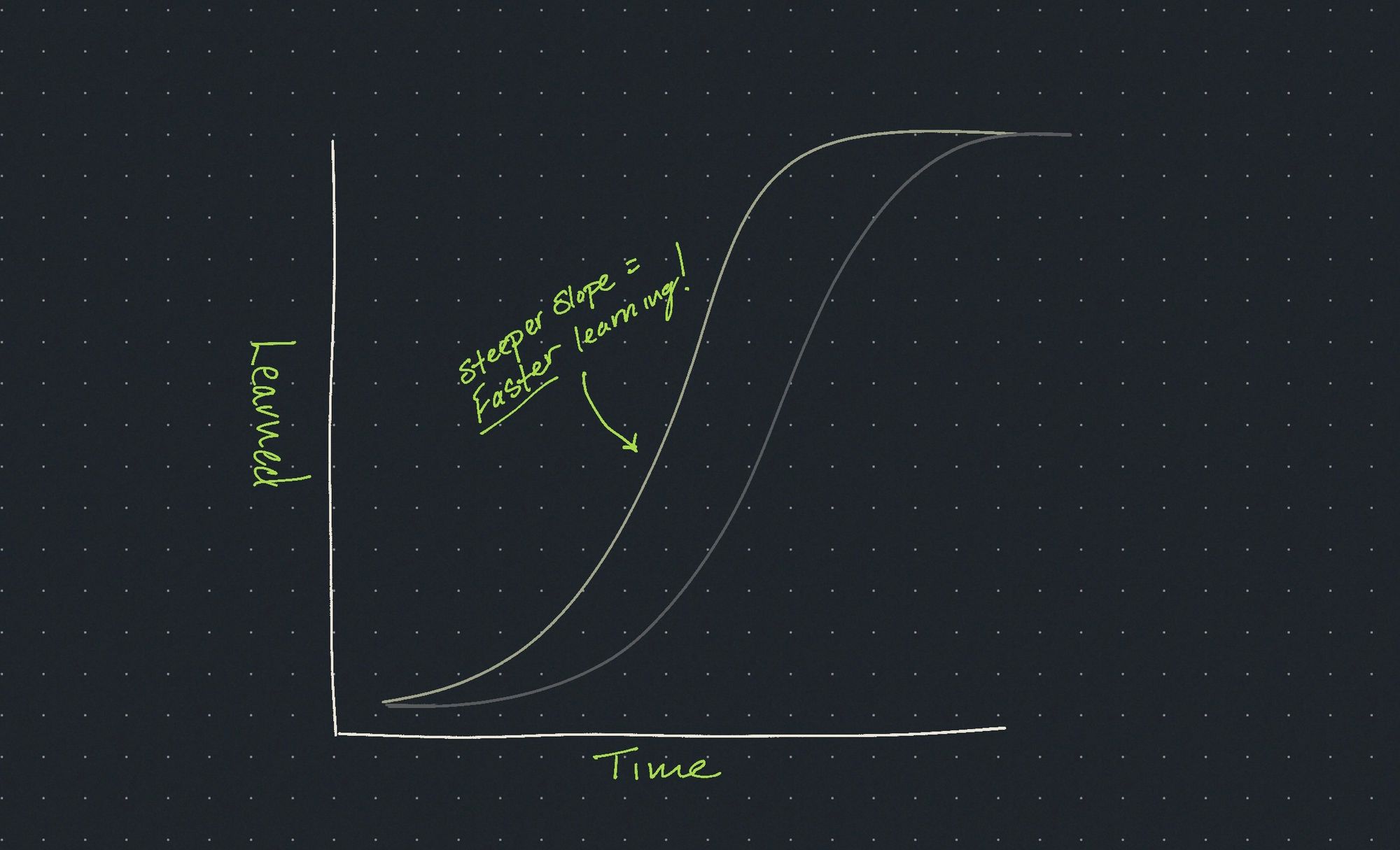
The "learning curve" is an often misunderstood concept. To say something is challenging, people often say it has a "steep learning curve," as if to imply learning that particular concept or skill is difficult. All a steep learning curve indicates is that gains toward mastery occur rapidly. The learning curve is simply a plot of success over time, not necessarily difficulty along the way.
Thorndike's research also developed a concept called connectionism or The Thorndike Effect; animals will do more of what they're rewarded for and less of what they're punished for, the essence of operant conditioning. For a child learning to walk, the reward is more efficient mobility, seeing the squirrels out the window better, and finally, reaching the knob for that cupboard. They learn how not to walk by the multiple attempts that lead to them falling. They learn that they need to shift their center of gravity slightly to make a loop, so they don't lose their balance. Many parents tend to make an error to tell their kids to "be careful" continually or "you're going to fall." While you don't want them walking off the back of the couch, you also don't want the lesson they learn to be "you can fall if you walk, so you should stop trying."
By falling, a child learns to avoid that approach to walking next time but not to avoid walking entirely. Take training wheels or a baby walker as examples; these tools help parents feel better by limiting the perceived risk of riding a bike or early walking but don't teach infants to do either correctly. Instead, they lead to "error-less learning," highlighted in Beginners. You aren't learning to ride a bike - you're learning to ride a bike with training wheels. You're no better prepared for the two-wheeled vehicle once the training wheels are gone.
Learning happens through a process called synaptic plasticity, the primary way our central nervous system learns. Here's the basic idea: the brain is a series of neural networks, a highway of connections between neurons. Certain connections are reinforced, and others are weakened. It's a process requiring trial and error to tell the brain what's essential. Imagine you have a network of neurons connected; they're all neurons to help you learn how to coordinate how to move your legs to push your body forward while upright.
At first, your muscles will fire somewhat randomly. Everly's first steps were rigid and off-balance. Though as she kept at it, her movements slowly became more natural-seeming. She could quickly take five steps, then 20, and soon walk from one side of the house to the other within a week or two. Her nervous system found a new way to do it just a little bit better through each trial. Her brain primed the neurons of that pathway through a mechanism called long-term potentiation. As her brain and spinal cord got the good news that what it was doing was working, the neurons that seemed best suited to tackling the issue were made more sensitive to the signal to move. Long-term potentiation forged the physical connections between adjacent neurons down that pathway more firmly together. These neurons want to get to work! Previous movement patterns that were tried but failed make neural connections less sensitive, making them less likely to fire on future attempts.
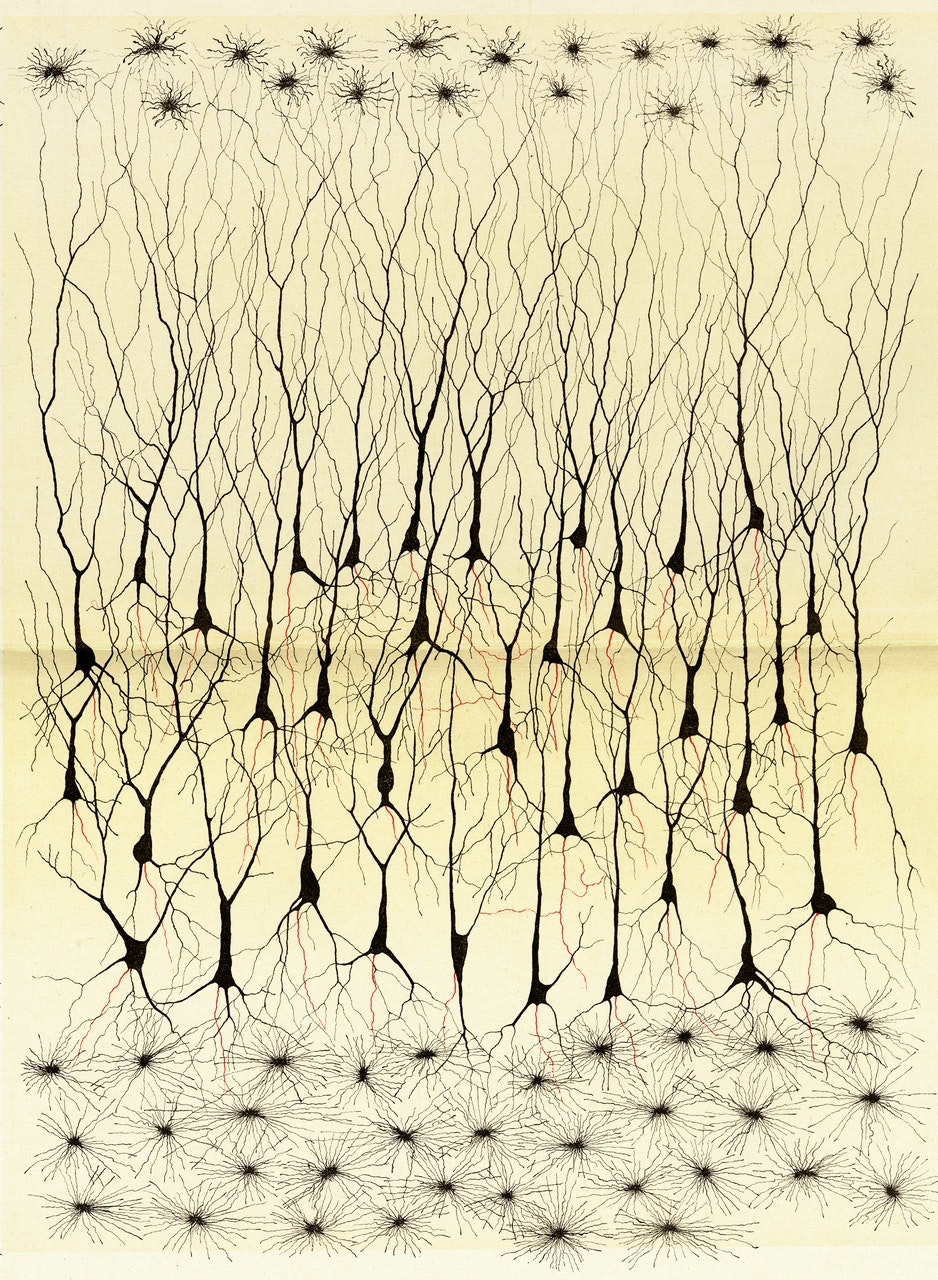
You can't walk for your child; you have to let them struggle through trial and error to figure it out independently. Infants who are carried a majority of the time learn less about their environment than infants who get around on their own. It's the same for adults when it comes to learning new skills too. Research at Columbia University has shown children and adults seem most apt to learning when they're especially confident in what they eventually find to be a wrong answer. In one study, adults were asked factually based questions and had to reply with a one-word answer. Researchers asked participants to rate how sure they were of the response they provided. They were shown the answers and then "retested" immediately after and again a week later. The most confident responses they got wrong were the ones that people typically most improved. The term used to describe this is called hypercorrection. There are a few theories about what might cause this, but the general idea is that committing a learner to an answer and providing feedback is the best way to help them learn. A learner will improve even if they are way off the mark, so long as they just commit.
Design for failure
In Beginner, Vanderbilt also describes a study looking at infants who watched adults learning how to get a toy out of a container. The adults were told to either easily retrieve the toy or show themselves struggling to get the toy out. Infants who watched the adults' struggle were more likely to stick with it when it was their turn to try and get the toy out. Infants who saw adults more easily do it were quicker to give up. Children who expected the path to be easy weren't as resilient. Failure is scary, though. No one wants to get the wrong answer or fall. We can't expect ourselves or our children to seek failure openly. Instead, we need to design systems around us that allow for failure to protect against harmful effects.
We all know infants are flexible and can withstand pretty good tumbles when first learning to walk. Look at how they're built!
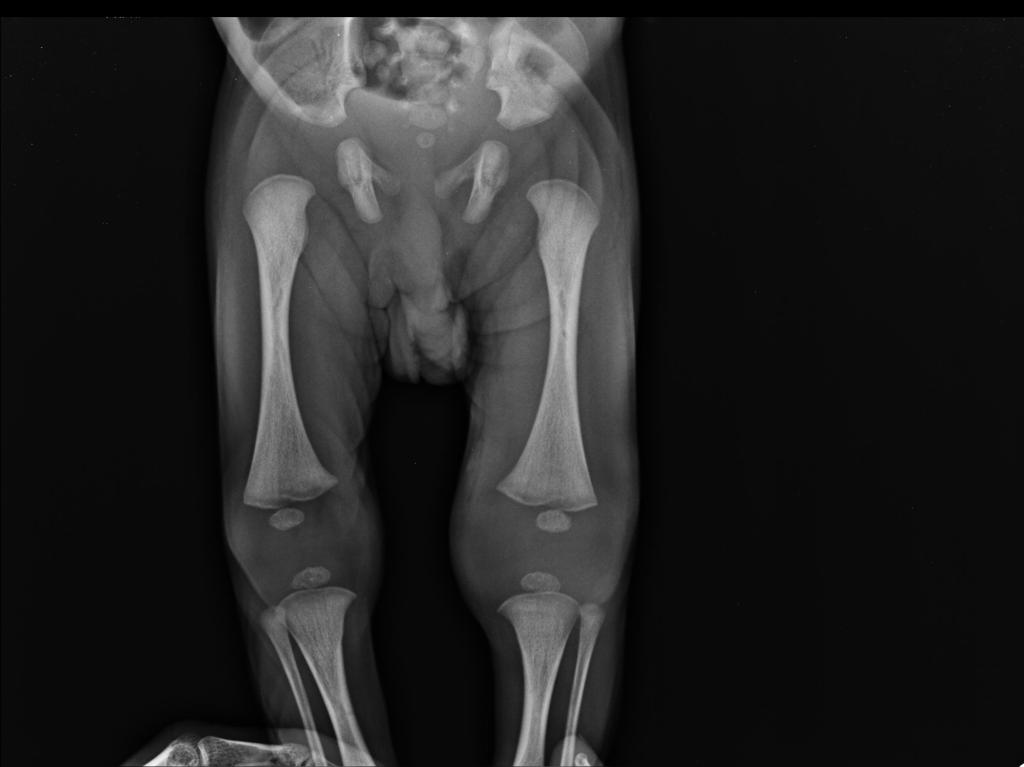
The physical system of an infant's legs and body are designed to deal with the expected falls - they're nothing but cushioning! It's a reminder that we need to build systems around us that help us deal with the failures and setbacks that we should expect. When you try to schedule your day to the minute, and you happen to get stuck at a railroad crossing, you put yourself into a position to lose. Or you try to guess where every dollar of your upcoming paycheck will go and then remember your car registration is due tomorrow - you're setting yourself up to fail.
The key is to engineer resiliency.
The setback isn't the thing that stops you in your tracks; it is the inability to deal with it and keep moving forward that stalls your progress. It's essential because failure is a learning opportunity. If you don't allow for failure, you won't be able to recalibrate and improve.
The Trick is to Fail When the Stakes are Low
That means to create early and test often. When learning a new topic in medical school, it was necessary to test yourself on the material right away. It's easy to convince yourself you understand something after reading it in a book or listening to a lecture. The only way to "fail" early was to take a quiz or answer a set of questions on the material. When you fail that test, it leads to the hypercorrection described earlier, which improves your learning and helps you prepare for when the stakes are higher - like when you take one of the three exams required to get your medical license. Another way to allow for safe failure is to create earlier prototypes of your idea. The concept of design thinking has become quite popular over the past several years. A core component of design thinking is creating early prototypes to create tangible artifacts of your thoughts and ideas. That can look like anything really - a mind map, storyboard, play-dough model, or an expertly 3D printed model. Doing this early on allows you to move past the brainstorming phase of an idea into a phase that allows for real-time feedback. There's no better time to learn that your vision for how to rearrange your workspace actually won't work because you didn't think of how the afternoon sun came through the window than before you went out to buy and build all that Ikea furniture.
Integrate prior experiences into new situations
From years of cognitive psychology research, we know that the more contexts a learner encounter an idea, the better they learn. Instead of simply calling to mind one particular example of something, a learner can create a network of mental models that can be applied to a new situation or problem they've never encountered. It's the reason grandpa seems so full of wisdom. It's not that grandpa has encountered every problem you've experienced; he's just encountered enough of them to build up an understanding of how the world works and can apply that to future difficulties more easily. Going back to Beginners, Vanderbilt describes how more experienced chess players are much faster and typically the best speed chess players compared to newer players. While beginners have to analyze every possible move every turn, experienced players focus only on relevant movements and can filter out the rest.
To understand this in a more nuanced way, I think it's essential to know how the brain functions to integrate information. We are organisms designed to perceive and recognize patterns in space and thought.
Futurist and author Ray Kurzweil describes this well in the book How to Create a Mind. He writes: "As each input from a lower-level pattern recognizer flows up to a higher-level one, the connection can have a “weight,” indicating how important that particular element in the pattern is. Thus the more significant elements of a pattern are more heavily weighted in considering whether that pattern should trigger as “recognized.”

Going back to the photo of the neurons above, you can see that each neuron can receive inputs from dozens of other neurons and then sends its signal to dozens of other neurons. Things get interesting when you look at the nervous system at the various levels Kurzweil refers to. We function in the world around us by taking in information through our different senses, creating a perception of the input in the brain. Then we form a response to them - whether it's pulling our hand off a hot pan, blocking the opposing player's shot, running from a snake, or sweating just before giving that big speech.
Each conceptual "level" of our nervous system has its system of recognizing patterns and generating meaning from them. For example, retinas cover the backs of your eyes - this is a collection of cells (sensors, really) called rods and cones. Rods are responsible for sensing black and white, and cones are responsible for sensing color (when I first learned this, I always remembered it by "c for color"). As light hits both sensor types, the nerve that connects your eyes to your brain (the optic nerve) immediately integrates the information. It's transformed from many light particles hitting your eye to a series of lines then sent to your brain. Once in the brain, multiple areas are responsible for further integrating the information from the eyes. We know this because people have studied this in kittens. If you put a pair of goggles made with striped lenses on a kitten when it's born, the cat's visual cortex in its brain becomes much more sensitive to light signals oriented in that direction.
Using prior experiences and memories, your brain interprets the series of lines coming from your optic nerves to be, "oh, that's a square" and then "oh, that's the way out of the room." To take action on that information, that interpretation is then sent to the motor cortices we talked about above. If it's something you've encountered before, the supplementary motor area digs into the memory banks to recall what you did last time to get through the door. If you've never seen a door quite like the one in front of you, the pre-motor cortex does its best to analyze the situation and use prior experience to develop a solution to this new problem. If it works, your brain will use its synaptic plasticity to remember that for next time. If not, you're sent back to the drawing board, in a state of hypercorrection, ready to adjust and carry on.
You can see that our brains work by recognizing patterns and integrating information based on feedback along the way. While we're all incredibly complex organisms, certain principles are true of all of us. We may all learn a bit differently; some things are true for each of us. Everly learning to walk is not that far off me learning how to sew someone's ear back together.
Failure is progress.